What are radiopharmaceuticals?
Radiopharmaceuticals are specialized drugs that use radioactive isotopes to diagnose and treat various medical conditions, most notably cancer. These drugs differ from other available treatment and diagnostic methodologies as they can precisely target and destroy cancer cells while minimizing damage to healthy tissues.
These innovative drugs utilize the unique properties of radiation to precisely target and manage disease, providing both therapeutic and diagnostic benefits.
A typical radiopharmaceutical consists of three primary components:
- Ligand: A ligand is most commonly a peptide, small molecule, or antibody that targets and binds to a specific biomarker or receptor expressed by the cancer cell. The ligand ensures the therapeutic agent is delivered directly to the cancer cells.
- Linker: The linker contains a chelating agent or chemical bonding site that links the ligand to a radioactive isotope. This component ensures that the radioactive isotope remains attached to the ligand until it reaches the target cancer cells.
- Radioactive Isotope: A therapeutic radioisotope emits high-energy particles, usually alpha or beta particles, that kill the cancer cell through DNA damage. The radiopharmaceutical is designed and tested to deliver the majority of its emissions to cancer cells, maximizing the therapeutic effect while minimizing exposure to surrounding healthy tissues.
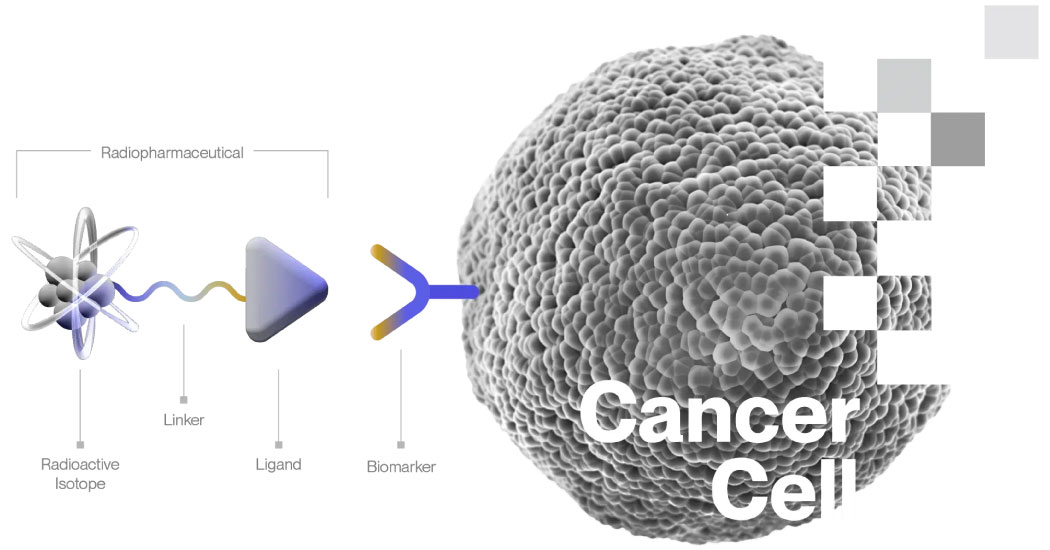
Therapeutic uses
Therapeutic radiopharmaceuticals are used to treat cancer by delivering targeted radiation to cancer cells. The ligand component ensures that the radioactive isotope binds specifically to cancer cells, minimizing exposure to healthy tissues. The radiation emitted from the isotope kills the cancer cells by damaging their DNA, effectively shrinking tumors and reducing cancer spread.
Lutetium Dotatate and Lutetium PSMA for the treatment of neuroendocrine tumors and prostate cancer are examples of therapeutic radiopharmaceuticals currently in use.
Diagnostic uses
For diagnostic purposes, the radioactive isotope emits positrons or gamma rays instead of alpha or beta particles. Imaging technologies such as PET (positron emission tomography) or SPECT (single-photon emission computed tomography) scans detect these emissions, allowing doctors to visualize the location and size of tumors within the body.
Theranostic applications
Theranostic radiopharmaceuticals offer both therapeutic and diagnostic capabilities, often through the use of biosimilar radiopharmaceuticals. A theranostic pair of radiopharmaceuticals, which are either similar or identical, uses one biosimilar molecule prior to therapy (on PET) and another biosimilar molecule for therapy and SPECT imaging.
It is important to note that while the overall structure of the radiopharmaceutical could remain the same, different isotopes or even different atoms are often used for therapeutic, diagnostic, and theranostic applications, which affect its properties.
For example, you could change the isotope from 64Cu to 67Cu or from 203Pb to 212Pb, but the molecule stays the same. But, if you change from 68Ga to 225Ac or to 117Lu, you’re changing the atom, and the structure of the radiopharmaceutical is different, albeit biosimilar.
How does radioligand therapy work?
When administered, these molecules circulate through the bloodstream, bind to cancer cells, and, when well-designed, remain there to exert their therapeutic effects. They only remain active for hours to days, after which they are inert.
Because the radiation from high-energy radioligand therapies travels only short distances, only the cancer cells specifically targeted by the ligand are damaged.
Importantly, if they do not find their target, they are designed to exit the body swiftly, reducing the risk of collateral damage, sparing healthy tissues, and minimizing side effects.
Another significant advantage of this technology is its ability to target and bind to individual cancer cells wherever they are in the body.
This means that even if the cancer has metastasized, spreading from the primary site to other parts of the body, the radioligand therapeutic can still target and treat these cells. This capability often allows the treatment of micro-metastases—small clusters of cancer cells that have spread but are too small to be detected by traditional imaging techniques.
Beta vs. alpha emitters
Radiopharmaceuticals use different types of radioactive emissions for their therapeutic effects, primarily alpha and beta particles:
-
- Beta emitters: These are small, negatively charged particles that travel several millimeters through tissue, causing moderate damage as they pass through. They effectively treat larger tumors but can slightly affect surrounding healthy tissues as the tumor shrinks.
- Alpha emitters: These are much larger particles that cause significant damage over a very short distance, making them highly effective at precisely killing cancer cells. Alphas can target and destroy even tiny clusters of cancer cells with minimal impact on surrounding healthy tissues.
Due to their size and destructive power, alpha emitters hold great promise for eradicating resistant cancer cells that survive other treatments. However, their development and supply chain are still evolving, making their widespread use more challenging than beta emitters, which have more established supply chains.
Radiopharmaceuticals vs. traditional treatments
From a patient perspective, radiopharmaceutical therapies offer a significant improvement over traditional cancer treatments. With radiopharmaceuticals, patients often avoid the severe side effects commonly associated with surgery, external beam radiation, hormonal therapy, and chemotherapy.
On a technical level, radiopharmaceuticals operate differently from traditional treatments. While chemotherapies and antibody therapies rely on specific chemical interactions with cancer cells to induce cell death, radiopharmaceuticals do not depend on these interactions.
Instead, the radiation emitted from the radioactive atoms kills the cancer cells regardless of the specific chemical signaling pathways involved. The only requirement is that the radiopharmaceutical be attached to the cancer cell (or, in some cases, near the cancer cell). This results in many new ways to design radiopharmaceuticals to target cancer that do not work for other therapies.
Bridging the gap from innovation to patient care
While scientific advancements have introduced innovative therapies like targeted radiopharmaceuticals, offering the possibility of more effective and less toxic cancer treatments, significant challenges remain in bringing these therapies to market.
The complexity of producing radiopharmaceuticals—from the need for specialized facilities for production and handling to the sophisticated supply chains for short-lived radioactive isotopes—presents hurdles that companies must overcome if they want their treatments to reach clinical settings.
That’s where Nucleus Radiopharma steps in as a crucial partner. As an end-to-end CDMO with a highly specialized team of industry experts, we’re building the clinical and commercial supply chains organizations bringing these drugs to market need.
Wondering how we can help you bring your radiotherapy to market? Learn more about our CDMO services.